-
-
-
-
-
- Reduced Pressure Differential
- Increased Drag
- Wake turbulence
-
-
- In-Flight
- Close to the Ground
- Along the Ground
-
- In-flight Separation
- Landing Separation
- Departure Separation
-
-
- Avoiding Vortices on Approach
- Avoiding Vortices on Takeoff and Landing
- Avoiding Vortices in Flight
- Avoiding Helicopter Outwash
-
- The Disadvantages of Winglets
-
-
What do you think happens to the air when an aircraft flies by?
The exhaust coming out of aircraft engines looks pretty dangerous, generating huge amounts of thrust and pushing back tons of hot air.
Would it surprise you to know that the most dangerous part of an aircraft’s wake is not the engine exhaust?
By far the strongest component of wake turbulence is the swirling air generated at the tips of the aircraft’s wings. This circular motion of air around the tip of the aircraft wing is called a wingtip vortex.
How Are Wingtip Vortices Formed?
When air flows over the aircraft wing, the shape of the airfoil creates low pressure above the wing and relatively higher pressure below the wing. The pressure differential results in a net force on the wing pushing it in the upward direction. This is called lift.
Air always flows from a region of high pressure to a region of low pressure. So, in the case of the aircraft wing, air tends to flow from below the wing to the upper surface of the wing.
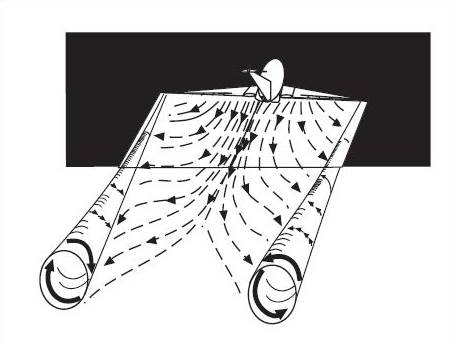
The movement of air happens at the wingtip, where the airflow moves out from under the wing, swirls around the tip, and tries to enter the low-pressure region at the top.
The circular motion that the air takes is called a vortex. Since it’s generated at the wingtip, it’s called a wingtip vortex.
The vortices aren’t just confined to the wingtip, though. The rotational movement of the air has inertia, and it continues to spread out behind the wingtip.
The vortices increase in diameter but decrease in intensity as time passes. These are called trailing vortices and they extend for quite a distance behind the aircraft.
Are Wingtip Vortices Visible?
Even though wingtip vortices are present whenever the aircraft is airborne, the swirling air usually doesn’t leave any visible signs of its existence. But, on very humid days, you might be able to see the core of the vortex trailing back from the wingtip.
This is actually a type of contrail!
Contrails are condensation trails; clouds that look like lines tracing the aircraft’s path across the sky. The most commonly seen contrails in aircraft are formed by the hot engine exhaust meeting the cold air.
Obviously, there’s no engine exhaust coming out from the wingtip. What’s causing the condensation there? The answer: pressure change!
The airflow inside the vortex core is spinning at a very high speed and has very low pressure. This reduces the temperature of the air inside. If the temperature drops below the local dew point temperature, the moisture in the air condenses rapidly, forming a small cloud right at the tip of the wing.
But if you’re looking at an aircraft flying high at cruising altitude, the contrails that you see are from the engines, not the vortices. The best chances of seeing contrails from wingtip vortices are when the aircraft is flying at low speeds and low altitudes on humid days.
These vortices are present at other locations where there are discontinuities in airflow as well. They’re present and occasionally visible at the ends of the aircraft flaps when they are extended for takeoff or landing.
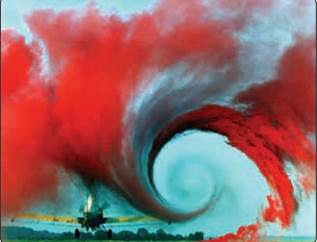
Another area where there’s a big pressure differential is at the fan blades of turbofan engines operating at a high power setting, such as on takeoff. If you look inside the engine intake during a takeoff run, you might see condensation fog inside the engine intake if it’s humid enough.
Propellor and rotor tips are another area where you might see contrails formed by tip vortices, although they look like a series of spirals instead of straight lines.
The best way to see the shape of the vortices is if you see the aircraft pass through some smoke or some cloud.
The image you see here is from a test conducted by NASA that had an aircraft fly through colored smoke. It shows the shape of the airflow behind the wing after the wing passed through it.
Wingtip vortices are a byproduct of lift. Once the wing stops producing lift, the vortices dissipate instantly. If you see an aircraft landing on a cold day when the vortices are visible, you’ll see that they disappear the moment the aircraft raises the wing spoilers.
Wing spoilers drastically reduce the lift generated by the wing. As soon as the spoilers rise, the wing stops generating enough lift to support the weight of the aircraft, and the vortices cease.
What’s Happening Inside the Vortex?
As seen from the aircraft’s tail, the vortex rotates in the anti-clockwise direction on the right wingtip and the clockwise direction on the left wingtip.
The rush of air travels downward from the underside of the wing as it rotates around the tip. This downward movement of air is called downwash. It’s the strongest right at the wingtip and decreases in intensity as you move along the span to the wing root.
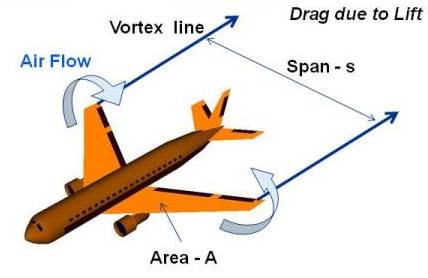
The downwash deflects the airflow behind the wing, pushing it downwards.
Why is this important?
It’s important because the lift is always produced perpendicular to the relative wind. Now that the relative wind is inclined, the lift does not point straight up and is in fact being generated at an angle.
This means that only part of the lift is now acting vertically, and some of it is being wasted by acting backward in the horizontal direction.
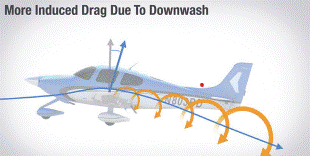
This horizontal component of lift is called Induced Drag. It’s called induced drag since it only exists as a consequence of lift. If you’re generating lift, you’re stuck with induced drag as well.
What Does the Vortex Strength Depend On?
As wingtip vortices are a direct consequence of lift, the same factors that influence lift generation also affect how strong the wingtip vortices are.
The strength of the vortices depends on the aircraft’s weight, the shape and span of its wing, and the speed of the aircraft.
It might seem counterintuitive, but the slower the aircraft is flying, the greater the strength of the vortex. That’s because you need to have a high Angle of Attack to be able to sustain flight at low speeds.
A higher angle of Attack is also needed when the aircraft is heavy since the wings need to generate more lift to support the increased weight.
The aircraft’s configuration also affects the vortex strength. When the aircraft is flying with the flaps down, slats or speed brakes extended, or gear down, it’s called being in a dirty configuration.
Flying ‘dirty’ will lead to a decrease in the vortex strength as the turbulence from the extended surfaces breaks down the vortex earlier. A clean configuration also needs a greater Angle of Attack as compared to a dirty configuration to generate the same lift for a given airspeed, which causes stronger vortices.
So basically, the wingtip vortices are more intense if the aircraft is heavy, slow, and is flying in a clean configuration.
Vortex size is also affected by proximity to the ground. If you’re flying low enough to be in ground effect, the vortex isn’t able to fully develop.
Strong winds are also a factor affecting vortices. They won’t directly change the intensity of the vortex, but they’ll help dissipate trailing vortices much quicker than still air.
Why Don’t We Want Wingtip Vortices?
Reduced Pressure Differential
The entire purpose of a wing is to develop a pressure differential that provides lift. But the high-pressure air leaking out into the low-pressure region reduces the pressure differential. The reduced pressure differential means that the wing is now generating less lift than it should.
Reduced lift for a given quantity of airflow means that you’ll need more airflow in order to achieve the amount of lift you need to bear the weight of the aircraft.
How do you get increased airflow?
If you fly faster, you’ll have more airflow over the wing, but doing so requires more engine power and consumes more fuel.
An alternate way of getting the lift you need is by flying with an increased angle of attack. This, again, means you’ll have to increase engine power.
Increased Drag
Moving air around is hard work! The vortices generated at the wingtips consume a pretty significant amount of energy to rotate the air. There’s no such thing as a free lunch in physics, so it’s your engines that have to work harder to account for this wasted energy.
Wake turbulence
These high-energy trailing vortices persist in the air long after the aircraft has passed by. This is bad news for any other aircraft unfortunate enough to meet them.
How Are Vortices Dangerous?
As high-intensity swirling air in the vortex can extend for quite a distance behind an aircraft, it poses a danger to any aircraft following or crossing its flight path. The trailing vortices are also called an aircraft’s wake, and the disturbed air is called wake turbulence.
Encountering light wake turbulence just makes the ride a bit bumpy. But if you fly closer behind an aircraft, you’ll be flying into a vortex that’s still fairly well developed.
This swirling air can induce a roll if it’s strong enough. The intensity of the rolling effect experienced depends on the preceding aircraft’s wingspan and the strength of the vortex.
When the aircraft encounters a vortex and it’s strong enough to induce roll, the pilot counters it by using the ailerons against the roll and tries to fly out of the wake as soon as possible.
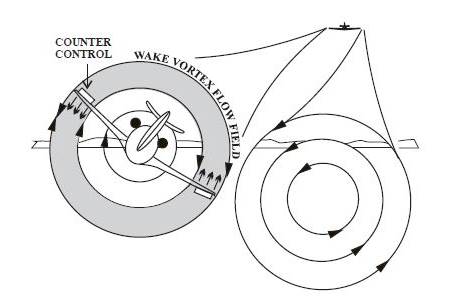
If the aircraft’s wingspan is long enough, its ailerons will extend beyond the vortex diameter, and counter control would still be possible.
In some cases, the induced roll could end up being stronger than the roll-control authority of the aircraft. That means that the ailerons are not large enough to counter the roll.
If you’re flying a plane with a short wingspan, you should do everything you can to avoid severe wake turbulence. Mass and inertia also matter. Wake turbulence that just shakes your coffee on an A380 might well be enough to flip a Cessna upside down!
Vortices that are more intense can even cause the following aircraft to cause structural damage, even to the point of breaking up the aircraft simply by flying too close behind an aircraft!
An example of how serious trailing vortices can be is an incident that happened in 2017 over the Arabian Sea. A small Challenger 604 business jet flying at Flight Level 340 passed one thousand feet below an Airbus A380 flying the same route in the opposite direction.
The Challenger got caught in the A380 vortices one to two minutes after it passed by. The vortices flipped the small jet upside down at least three to five times before the pilots managed to regain control.
This is one of the reasons why passengers are advised to remain seated with their seatbelts on even when the seatbelt sign is turned off.
There have been numerous incidents of passengers and cabin crew suffering injuries ranging from mild to severe after their aircraft bounced around after getting caught in someone else’s wake.
The greatest chances of encountering an uncommanded roll are if the following aircraft is flying on a heading generally aligned with the preceding aircraft.
Vortex Behavior
The tricky thing about wake turbulence and wingtip vortices is that they are invisible. They can only be avoided by predicting their behavior.
The pilots need to visualize the wake from other traffic and ensure that they are flying far enough behind for the vortices to dissipate from the leading aircraft.
Pilots need to know how vortices behave and dissipate so that they can predict their movement and avoid catching wake turbulence.
In-Flight
So what happens to the vortices after the aircraft has flown by?
The vortices remain suspended in the air, spaced less than a wingspan apart. They’re affected by wind, but since they still possess a high level of energy, they’re able to maintain their shape. The vortex, as a whole, drifts along with the wind.
Pilots can find out the prevailing winds aloft such as from the NOAA Aviation Weather Center and predict the vortices’ direction and rate of drift behind the preceding aircraft’s flight path.
The stronger the wind is, the farther away the vortices will drift from the flight path.
When flying behind a large aircraft on the same route, the pilots can adjust their course upwind to avoid getting caught in the wake.
As time passes and the vortices lose energy, they sink at a rate of several hundred feet per minute as they dissipate.
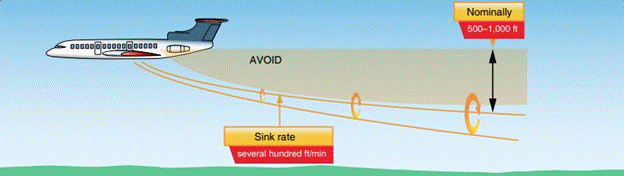
If there’s significant atmospheric turbulence around, it’ll help dissipate them faster. The flipside to this is that on calm days with still air, wake vortices will be hanging around for a longer period of time.
So if you’re crossing an aircraft’s wake, your best chance of avoiding wake turbulence is to fly at or above its flight path, also keeping the prevailing wind in mind.
Close to the Ground
The vortex movement changes when they’re within a couple of hundred feet to the ground. If they’re generated by a large aircraft flying at one or two thousand feet, the vortices won’t have deteriorated entirely by the time they sink to the ground.
As the swirling air strikes the ground, the vortex is pushed laterally along the surface. Pilots need to keep this behavior in mind as they’re likely to encounter these vortices while they’re on approach or during departure.
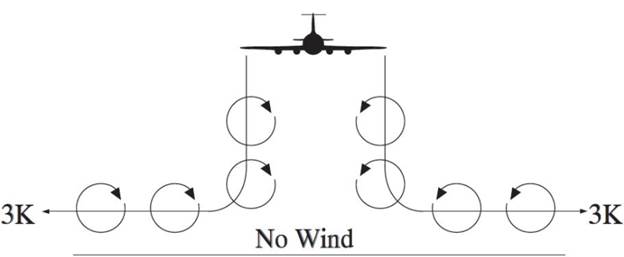
This lateral movement takes place at a speed of about two or three knots.
As at higher altitudes, the vortex movement near the ground is affected by crosswinds. The downwind vortex can be pushed along by the wind farther than expected, while the upwind vortex has the wind acting against it.
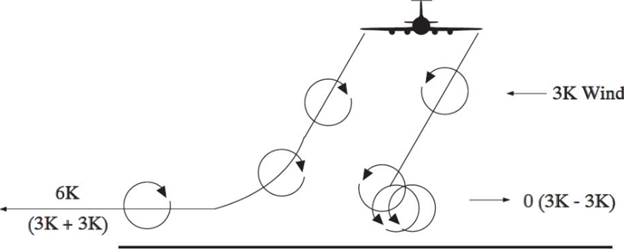
The most dangerous vortex movement is caused by a tailwind. After one aircraft lands, the tailwind pushes the vortices forward into the runway’s touchdown zone. The next aircraft to attempt a landing is likely to encounter the vortices right at the critical moment of touchdown.
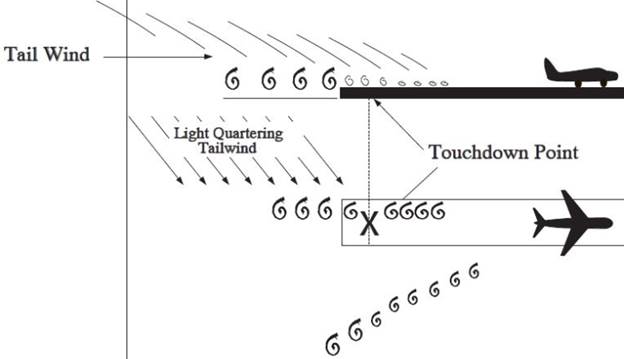
This lateral movement of vortices is important to know for pilots flying into airports with parallel runways. The FAA only allows simultaneous parallel operations on runways that are far enough for it to be safe for the trailing aircraft. Here are some examples of the FAA’s guidelines for some airports with parallel approaches.
Along the Ground
Once you’re flying along the surface of the ground, the airflow dynamics change quite a lot. The aircraft seems to float on a cushion of air and doesn’t seem to want to land.
This phenomenon is called Ground Effect. Ground Effect happens only when flying at a height of a few feet above a surface and is caused by the interaction of wingtip vortices with the landing surface.
When the aircraft is flying low enough, the vortices don’t have enough room for the air to flow down past the wingtip.
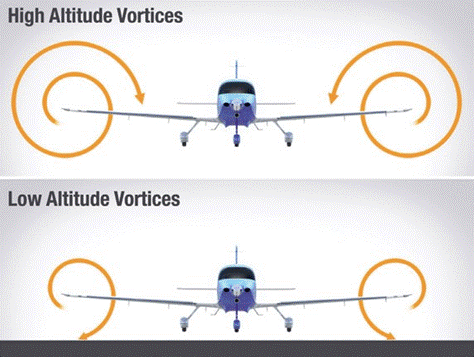
Note that the ground effect doesn’t eliminate the vortex entirely. The airflow near the wingtip is still able to curve around and make a vortex.
It’s the airflow further away from the wingtip that doesn’t have enough room to make it all the way around the tip. The resulting wingtip vortex is smaller and less intense.
It is still able to move laterally, and it’s even possible for vortices to bounce off of the ground and rebound up to a height of twice the aircraft’s wingspan.
A reduced vortex also causes a reduction in the induced drag as well as an increase in the pressure differential between the upper and lower surface of the wing.
The wing is suddenly able to generate a lot more lift, and as a result, it feels like the aircraft wants to float along the surface.
Ground effect is only noticeable at heights less than the aircraft’s wingspan. The reduction in induced drag is only around one percent at the height of one wingspan, but rises to more than 23 percent at the height of one-fourth of the wingspan!
Aircraft Classes
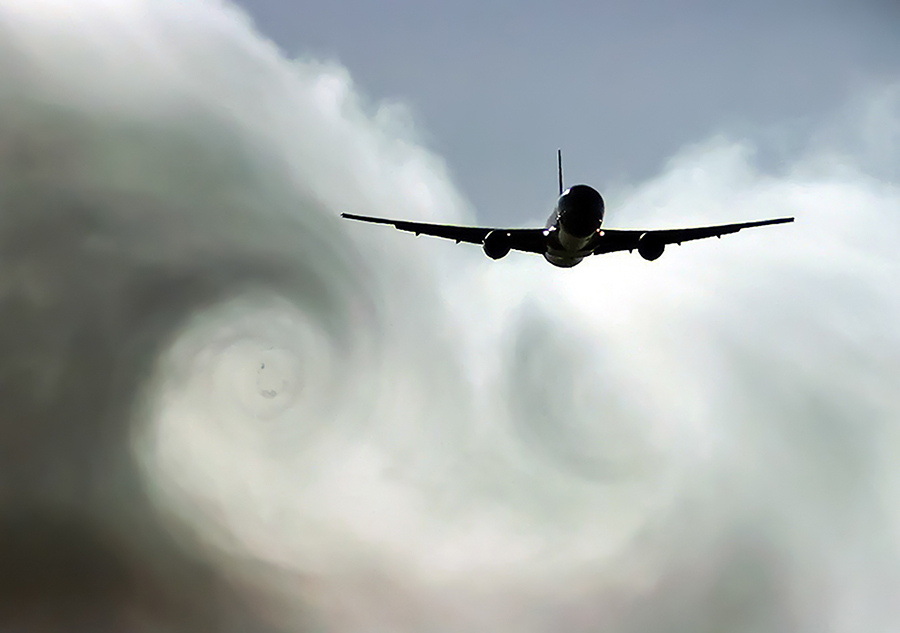
As vortex size and intensity depend largely on the mass of the aircraft, aircraft are classified into different categories depending on the amount of wake turbulence they generate.
Air Traffic Control uses this classification to separate aircraft and ensure that their wake turbulence does not affect other aircraft.
These rules are based on the fact that generally, larger and heavier aircraft generate intense vortices.
Similarly, the effect of flying through wake turbulence is felt more severely by light aircraft, whereas heavy aircraft have so much inertia that even moderately strong wake turbulence doesn’t pose a danger to them.
The FAA currently uses aircraft weight to classify aircraft. The list of aircraft types are:
- Small – Aircraft weighing less than 41,000 pounds maximum certified takeoff weight.
- 757 – Boeing 757s.
- Large – Aircraft weighing more than 41,000 pounds but less than 300,000 pounds at takeoff.
- Heavy – Aircraft weighing 300,000 pounds or more at takeoff.
- Super – Airbus A380-800 and Antonov An-225.
As the danger of wake vortices is greater from large aircraft, they append their callsign with the word “Heavy” so that other pilots on the frequency pay more attention to their calls and are aware of their position and route.
Pilots of the A380 and AN225 append the word “Super” for the same reason.
The Boeing 757 is classified in a separate category altogether. It’s not as big as a large aircraft, but the wake turbulence it was generating turned out to be significantly more severe than other small aircraft. ATC treats it as a heavy aircraft and also advises small aircraft if the preceding aircraft is a 757.
The FAA has established different rules for in-flight separation, approach separation, and arrival and departure separation.
In-flight Separation
When flying at the same altitude or at less than 1000 feet below, regulations require:
- Small aircraft behind 757 – 4 miles of separation.
- Small or Large aircraft behind a Heavy – 5 miles of separation.
- Small aircraft behind Super – 8 miles of separation.
- Large aircraft behind Super – 7 miles of separation.
- Heavy aircraft behind another Heavy – 4 miles of separation.
- Heavy aircraft behind a Super – 6 miles of separation.
Landing Separation
The critical period of approach and landing is also regulated by the FAA for adequate separation:
- Small landing behind a Large aircraft – 4 miles of separation.
- Small landing behind a Heavy – 6 miles of separation.
This distance is measured when the preceding aircraft is over the runway threshold.
Departure Separation
The FAA regulates departure separation according to the time interval after the leading aircraft takes off.
- Waiting for three minutes behind a departing Super aircraft.
- Waiting for two minutes behind a departing Heavy or a 757.
These intervals are necessary when the leading aircraft has taken off from the same runway, from a parallel runway less than 2500 feet apart, or a crossing runway with crossing flight paths.
Smaller aircraft often take off from a runway intersection instead of using the full runway length.
A Small category aircraft taking off from a runway intersection behind a Heavy, a Large aircraft, or a 757 needs to wait for at least three minutes.
Remember that wake turbulence is largest when the aircraft is heavy, slow, and in clean configuration. As this is the case with aircraft that are departing, the rules generally don’t allow ATC to waive off these intervals.
In some cases, the pilots may request an earlier departure, depending upon the rules laid down here.
What’s RECAT?
The current system for classifying aircraft is based on weight, which, as described earlier, is not the only factor affecting the intensity of wingtip vortices.
The new classification is based on a combination of aircraft weight, approach speed, and wingspan to give a more accurate representation of the wake produced by the aircraft.
The new classification system is being developed with the Wake Turbulence Recategorization program (RECAT) and is being implemented in phases.
Future phases of RECAT will include dynamic pairing, which means it’ll take live weather into account while calculating required spacing!
Since the current system relies on a generalized idea of vortex intensity and bases it only on aircraft weight, it is not as precise as it could be and has all its separation rules written in a conservative manner for safety.
With RECAT using more precise estimates of vortex strength for each aircraft, the spacing requirements between aircraft can be reduced.
These estimates are calculated for each possible pair of aircraft, which means that it accounts for aircraft that generate exceptionally strong vortices, and spaces them safely.
This allows airports and flight routes to handle more aircraft and improves the efficiency of the entire air traffic system without compromising safety.
This system has the following categories:
- Category A – Airbus A380 and Antonov An-225.
- Category B – Pairwise Upper Heavy aircraft, including B777, B787, and A330.
- Category C – Pairwise Lower Heavy aircraft, including B767 and C-17.
- Category D – Non-Pairwise Heavy aircraft, including B743 and older heavy aircraft.
- Category E – B757, including the -200 and -300 variants.
- Category F – Upper Large aircraft including Airbus A320 and Boeing 737.
- Category G – Lower Large aircraft. ATRs, Dash 8s, large business jets.
- Category H – Upper Small aircraft with a maximum takeoff weight of more than 15,400 pounds up to 41,000 pounds. This includes most smaller business jets.
- Category I – Lower Small aircraft with a maximum takeoff weight of 15,400 pounds or less. This includes single or multi-engine prop planes including Cessnas and Pipers.
RECAT is currently operating at a few airports in the US and is continually being implemented in more airports.
How to avoid wingtip vortices?
In addition to following the separation rules laid out by the FAA, there are also additional methods pilots can use to decrease their chances of being caught out by an errant vortex.
Avoiding Vortices on Approach
When you’re flying under air traffic control guidance, ATC does direct traffic to provide enough spacing between aircraft for the vortices to dissipate.
Not only is the lateral distance between two landing aircraft important, but since the vortices spread out over time, the trailing distance between the two aircraft is also regulated.
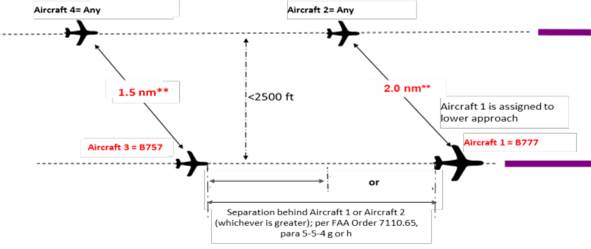
But if you’re flying visually, at an uncontrolled airfield, or when ATC asks you to maintain your own separation, it’s up to the pilot to know how much distance to put between the preceding aircraft.
Pilots have a number of ways to increase their distance from the preceding aircraft. ATC may ask the pilot to slow the aircraft down earlier in the approach to allow time for the vortices to dissipate.
The pilot may have to extend the landing gear and add more flaps to slow the aircraft down, but the aircraft is limited by its stall speed.
It’s also more dangerous to enter a vortex at slow speeds since recovery is difficult when you have less energy at your disposal.
If ATC notices that there won’t be enough separation even after slowing down, they’ll ask the trailing aircraft to pause the approach and enter a holding pattern.
Small aircraft can fly dog-legs or fly in an S-shaped pattern to increase their distance from the preceding aircraft.
Pilots of Large, Heavy, and Super aircraft need to fly their approaches exactly on the glide path, and never above it. If they were to fly above the glide path, their wake would sink down and intercept the next aircraft to fly along the glide path.
Avoiding Vortices on Takeoff and Landing
Takeoff and landing are critical sections of flight. The aircraft is in close proximity to the ground, and there’s not a lot of time or altitude available to regain control of the aircraft if you find yourself in a vortex.
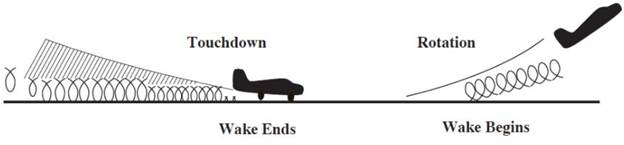
While ATC does space departures and arrivals to allow time for the wake to dissipate, the final responsibility for wake avoidance lies with the pilot.
The most reliable way for avoiding wake turbulence is waiting till it dissipates.
But for operations at busy airports, or at uncontrolled airfields, the pilots can use these guidelines for minimizing the chances of a wake encounter.
- If you’re landing after an aircraft that is larger than yours, fly at or above the glidepath. If the runway and the aircraft are fitted with an ILS system, it’ll show the glidepath on the instruments and you can use that as a reference to adjust your approach accordingly. Alternatively, you can also use the VASI lights to help you out.
- Note the point on the runway where it touched down and make sure to land beyond it. You’ll see a puff of smoke as its tires come into contact with the ground. If there’s enough runway length available, you can land beyond that point to ensure your entire approach and landing is clear of the wake.
- Watch out for drifting vortices if there’s an aircraft ahead of you landing on a parallel runway. Crosswinds can push the downwind vortex into your approach path. Again, it’s safer to stay above the leading aircraft’s flight path and land beyond its touchdown point.
- If you’re landing behind a larger aircraft that’s departing off of the same runway, take note of its point of rotation and make sure to land well before that point. You should also note its direction of travel after it takes off. If you end up having to go around, you wouldn’t want to cross its wake.
- If you’re departing behind a large aircraft, you need to be able to rotate before the point on the runway where it took off. Not only that, but you also need to climb at a faster rate than the lead aircraft.
- If you’re landing or departing behind an aircraft that just executed a missed approach, you’re in danger of encountering laterally moving vortices. In this case, it’s best to just wait a couple of minutes for the vortices to dissipate.
Avoiding Vortices in Flight
Air Traffic Control doesn’t just make sure aircraft don’t collide with each other, they also direct aircraft to avoid each others’ wake turbulence. Generally, they’ll be using procedures for separating IFR traffic, but they may also issue guidance for VFR traffic.
VFR traffic flies under the “See and Avoid” approach. It’s the pilot’s responsibility not just to avoid collisions with other aircraft, but also to anticipate their wake turbulence and avoid that as well.
A little extra situational awareness doesn’t hurt though, and if ATC notices your flight path crosses someone else’s wake, they advise you of the position, speed, direction of flight, altitude, and aircraft type to give you a better idea of where and how intense their wake might be.
ATC can also remind you to exercise “CAUTION – WAKE TURBULENCE” and you’d be well-advised not to take that warning lightly.
An absence of ATC warnings doesn’t mean you can assume your flight path to be clear of wake turbulence. Pilots are always responsible for the safe operation of the flight. If there’s any doubt about adequate separation, pilots should always play it safe and ask ATC about traffic in their area.
If you’re flying VFR, you’ll be flying at thousand-foot altitudes plus five hundred feet. Other VFR traffic will have a vertical separation of one thousand feet from you, and IFR traffic will be flying five hundred feet above or below you.
Avoiding Helicopter Outwash
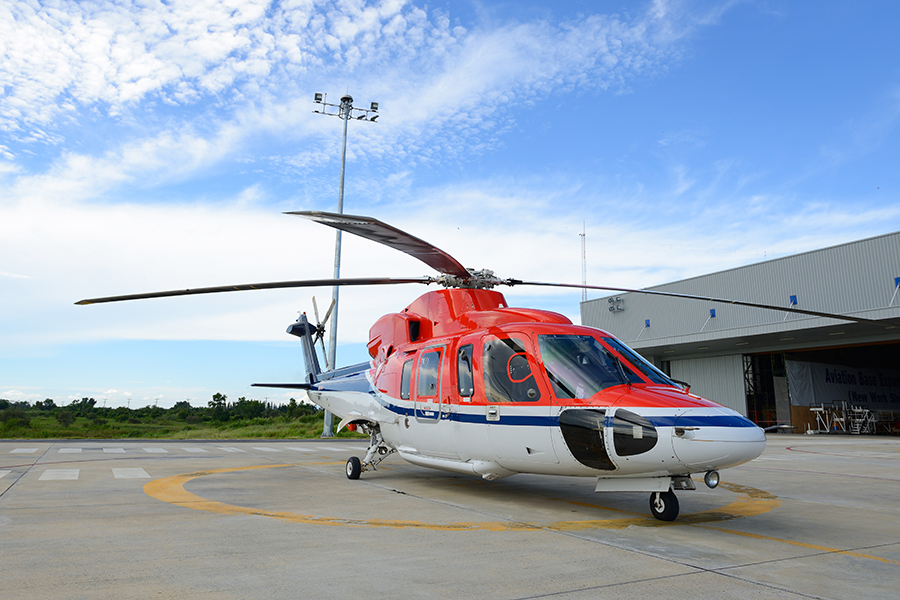
The rotors of a helicopter also generate vortices at their tips. When they are hovering close to the ground, downwash from the rotor bounces off from the ground and spreads outward.
These vortices can be dangerous within three rotor diameters, so watch out for helicopters that are hovering or hovering taxiing; particularly those that are upwind from a runway.
When they are flying forwards, helicopters produce trailing wake vortices that are very similar to those from an aircraft.
Remember that helicopters fly a lot slower than aircraft. The wake they generate during forwarding flight can be a lot stronger than you might expect.
In 2009, a small Piper Cherokee aircraft was following a Sikorsky S76 helicopter on approach. Both aircraft were classified in the ‘Light’ category for wake turbulence.
Even though the Piper was a mile behind the helicopter, it got caught in the vortices and was flipped upside down. It hit the ground inverted and resulted in serious injuries to the pilot.
How to Reduce Wingtip Vortices?
Given that wingtip vortices create the drag, reduce lift, and pose a danger to other aircraft; aircraft manufacturers developed several methods to reduce vortex generation.
Take a look back at what happens to vortices when the aircraft is flying in ground effect. The proximity of the ground impedes the airflow around the wingtip, and the effect is very noticeable.
Aircraft manufacturers attach barriers on the tips of the wings to achieve the same result: preventing airflow around the wingtip.
These wingtip devices are generally referred to as winglets, although some manufacturers give them their own names for branding. Airbus added a winglet on the top as well as on the bottom part of the wingtip on the A300, A310, A320, and A380; and called them wingtip fences.
Airbus also designed new winglets for the A320 and calls them ‘Sharklets’. Boeing added new winglets on their 737 jets and calls them ‘Split Scimitar winglets’.
Manufacturers of smaller aircraft even added fuel tanks at the wingtip. This allowed them to make the wing itself thinner, while the tip tank helped block the wingtip vortex too.
Another method of reducing the flow of air is by making a tapering wingtip. This smooths the airflow, prevents an abrupt pressure differential, and reduces the intensity of the vortex.
The Disadvantages of Winglets
The downside of adding winglets and tip tanks is that they increase weight and act as an additional source of parasitic drag.
A set of Boeing 737 winglets weigh more than 250 pounds. The weight of the winglet bends the wing down and adds a significant amount of stress to the wing. This requires the wing to be strengthened, which adds even more weight.
The gain in fuel efficiency more than makes up for the parasitic drag created by the winglets, which is why they are installed on most aircraft being built today.
Winglets don’t come cheap though, and they are only really effective during cruises. This makes them economically viable mostly for long-haul aircraft.
Unfortunately, their effectiveness at reducing wake turbulence is very limited during the times when it’s needed the most: The wake turbulence is largest when the aircraft is flying slowly, and winglets are least effective at low speeds.
The case against tapered wingtips is that it greatly increases the aircraft’s wingspan. That makes it harder for the aircraft to fit into hangars and airport gates. A solution to this problem is brought by Boeing in the 777X aircraft which is fitted with foldable tapered wingtips.
Can Wingtip Vortices Be Useful?
Wingtip vortices aren’t all bad news though. In some cases, they can actually be beneficial, and no one knows this better than birds! Have you ever wondered why birds fly in a V-shaped pattern? They’re actually using each other’s wingtip vortices to make flying easier!
Air in the vortex moves upward outboard of the wing and comes back down inboard to complete the vortex. Birds can feel the rising air, and they position themselves slightly behind and outboard of the preceding bird’s wing, exactly in the place where the air is rising in the vortex. The result? A free lift!
Since every bird apart from the leader is flying in rising air, they don’t have to work as hard to generate enough lift to support their weight. This saves them energy and allows them to fly for even longer distances.
Do pilots fly in formation to save fuel too?
At the moment, no.
As we’re not able to ‘feel’ the air, it’s not easy to figure out the best position to take relative to the other aircraft in the formation. Secondly, formation flying isn’t that easy and is a bit risky.
For small aircraft, the airflow and the turbulence are too chaotic to fly close enough to take advantage of the upwash.
But large commercial aircraft could be flying along like geese in the near future. In November 2021, Airbus conducted trials with two A350 aircraft flying in formation to see how much fuel they could save on a transatlantic flight making use of the upwash generated by the lead aircraft.
As the A350 is a large aircraft with a massive wingspan, the vortices it creates are relatively stable and last long enough for another aircraft to follow along at a safe distance while still being able to take advantage of the upwash.
The test flight showed a fuel saving of up to 5 percent and a reduction of more than 6 tons of Carbon Dioxide emissions, even though the aircraft were flying a mile and a half apart!
Conclusion
Invisible but ever-present, wingtip vortices can take even experienced pilots by surprise. With the help of ATC guidance, improvements in technology, and a better understanding of vortex behavior, the danger from wingtip vortices can be reduced, but never fully eliminated.